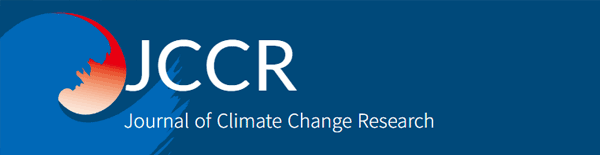
Microbial Community Structure of the Active Layer Soil from Resolute, Canadian High Arctic
Permafrost is frozen soil below 0℃ for two or more years. Surface of permafrost is called as active layer that seasonally thaws during the summer. Although the thawing of permafrost may deepen the active layer and consequently increase the microbial activity, the microbial community structure in this habitat has not yet been well described. In this study, we presented bacterial and archaeal diversity in the active layer soil from Resolute, Canada using pyrosequencing analysis. The soil sample was collected from the surface of the marsh covered with moss and Carex. A total of 7,796 bacterial reads for 40 phyla and 245 archaeal reads for 4 phyla were collected, reflecting the high diversity of bacteria. Predominant bacterial groups were Proteobacteria (37.7%) and Bacteroidetes (30.0%) in this study. Major groups in Archaea were Euryarchaeota (51.4%) and Thaumarchaeota (46.1%). Both methane producing archaea and consuming bacteria were detected in this study. Although it might be difficult to characterize microbial community with only one sample, it could be used for the basis of assessing the relative importance of the specific groups with a high resolution on the bacterial and archaeal community in this habitat.
Keywords:
Arctic, Active Layer, Archaea, Bacteria, Microbial Community Structure1. Introduction
Active layer, the surface layer of permafrost, provides a potential indicator of climate change (Guglielmin, 2004), because the active layer depth is sensitive to changes in air temperature. According to the prediction of current models (Lawrence and Slater, 2005), the near-surface permafrost thawing, that is, active layer formation will be accelerated during the first half of the 21st century. Thawing areas of permafrost are associated with increased fluxes of CH4, CO2 and N2O (Zimov et al., 2007). And there is a concern that this thawing will be caused by large-scale emissions of methane due to increased microbial activity, linking to the feedback loop on global warming (Christensen et al., 2004). However, compared to the study of permafrost, the study of microorganisms in active layer is very limited (Zhou et al., 1997; Liebner et al., 2009; Martineau et al., 2010; Yergeau et al., 2010).
DNA sequencing-based microbial community studies have been widely propagated by the invention of pyrosequencing, which produces millions of sequences in a single run (Ronaghi, 2001). Pyrosequencing generates millions of sequences in a single run excluding the time-consuming step of constructing clone libraries. Therefore, tremendous sequence information in a sample can be obtained, from which deep insights of microbial community can be gained. The soil microbial community structure of active layer is essential to understand microbial processes in the emission of CH4 and CO2 by permafrost melting. Therefore, we analyzed the bacterial and archaeal community in the active layer soil through pyrosequencing as an initial step to understand the microbial contribution to the greenhouse gas production in permafrost ecosystem.
2. Materials and Methods
2.1 Sampling and DNA Extraction
Soil sampling was conducted in Resolute on Cornwallis Island in Nunavut, Canada (74°41′N, 94°54′ W) on 6th August 2009 (Fig. 1). Study area can be identified as a polar desert constituted of limestone and dolomite pebbles in a matrix of sand and silt with pH 7.0 to 8.0 (Cruickshank, 1971). A mean air temperature and annual precipitation of Resolute is -16.4℃ and 161 mm, respectively (En-vironment Canada, 2014). The soil sample was collected from the surface of the marsh covered with moss and Carex (Fig. 1). Soil was collected by using of 50 mL conical tubes from active layer (Fig. 1), and fixed in RNAlater (Ambion). Genomic DNA was extracted using the UltraClean Soil DNA kit (Mo-Bio), following the manufacturer's guidelines. Concentration and quality of the gDNA was checked by electrophoresis on 0.8% agarose gels.
2.2. Pyrosequencing
In order to obtain amplicons of 16S rRNA gene fragment of the appropriate size and sequence variability for 454 pyrosequencing, primer sets of 27f (GAG TTT GAT CMT GGC TCA G) and 518r (WTT ACC GCG GCT GCT GG) for bacteria (Weisburg et al., 1991) and 21f (TCC GGT TGA TCC YGC CGG) and 516r (GGT DTT ACC GCG GCK GCT G) for archaea (DeLong, 1992; Qian et al., 2011) were chosen, which contained the hypervariable regions from V1 to V3. Three independent PCR reactions were carried out for each sample. PCR was set up in a total volume of 50 μL containing 10 ng of DNA as a template, 20 pmol of each primer, 1×PCR buffer (10 mM Tris-HCl; 15 mM MgCl2 50 mM KCl, pH 8.3), 10 nmol of each dNTP and 1 U of Taq polymerase (Roche). The amplification consisted of an initial denaturation at 94℃ for 5 min, followed by 10 cycles at 94℃ for 30s, 60℃ to 55℃ with touch-down program for 45s and 72℃ for 90s and additional 25 cycles at 94℃ for 30s, 55℃ for 45s and 72℃ for 90s. The size of amplicons was checked by electrophoresis in 2.0% agarose gel stained with ethidium bromide. The triplicates were pooled together and purified using Qiaquick PCR purification kit (QIAGEN). The purified products were quantified using a spectrophotometer (NanoDrop Technologies, Rockland, DE, USA) and mixed in equivalent proportions. Pyrosequencing was performed by ChunLab, Inc. (Seoul, Korea) using a 454 Genome Sequencer FLX (Roche).
2.3 Bioinformatic Analysis
Sequencing reads were separated by barcodes, and 2 bp of linker and forward and reverse primers were trimmed. Sequencing reads with two or more Ns and short reads (the number of base calls of Q<20 is less than 200) were omitted from the dataset. Raw flowgrams (sff files) were filtered. Additionally, noise and chimera sequences were omitted. Individual sequencing read was compared to sequences in the EzTaxon-e database (http://eztaxon-e.ezbiocloud.net) (Kim et al., 2012). Five sequences with the highest hit scores were searched to sequence similarity using global sequence alignment. The best match with the highest sequence similarity value was used for taxonomic assignment of query sequences. We used the cutoff values for taxonomic assignment of each read; species ≧97%; 97% > genus ≧ 94%; 94% > family ≧90%; 90% > order ≧85%; 85% > class ≧80%; 80% > phylum ≧75% (Stackebrandt & Goebel, 1994). When the similarity value is below the cutoff, the read is assigned as unclassified group. For the analysis of microbial diversity, OTUs were defined by using the CD-HIT program (Li and Godzik, 2006). Species richness/ evenness was estimated using Chao1, ACE, Shannon and Simpson indices by using the MOTHUR program v.1.8.0 (Schloss et al., 2009). To compare the diversity of the 16S rRNA gene sequences in this soil, number of OTUs and statistical richness estimate of Chao1 and ACE were performed using ≦ 3% distance cutoff at DNA level to define OTU, which is most frequently used for 16S rRNA gene (Hughes et al., 2001). The results were visualized by CLcommunity software provided by ChunLab (http://www.chunlab.com).
3. Results and Discussion
We investigated the microbial community structure from permafrost-affected soil in Resolute, Canada. We obtained a total of 8,393 bacterial reads and 1,111 archaeal reads with an average length of 457 bp (a range from 300 to 530 bp) from the pyrosequencing of PCR amplicons (Table 1). Among these sequence reads, 597 bacterial reads and 866 archaeal reads belonged to eukaryote. Since we are only concerned with prokaryotic cell affiliated reads these eukaryotic reads were removed in the final dataset for further analysis. Hierarchical taxonomic classification of refined 7,796 bacterial and 245 archaeal reads were performed on the basis of the alignment of reads to the sequence of EzTaxon-e Database (Table 1). Although the number of read analyzed was uneven, when we compared the bacterial and archaeal communities through diversity indices, bacterial abundance and diversity were significantly higher than that of Archaea (Table 1). This difference in diversity/evenness between the bacterial and archaeal communities corresponded to other studies (Hansel et al., 2008; Steven et al., 2008).

Summary of bacterial and archaeal sequences including the number of total and target reads, estimated number of OTUs, richness by Chao1 and ACE and evenness by Shannon Simpson indices using MOTHUR program by ≦3% distance cutoff value
Various bacterial groups were revealed in this study. Bacterial sequences were hierarchically classified into 40 different phyla; Proteobacteria, Bacteroidetes, Firmicutes, Cyanobacteria, Actinobacteria, Chloroflexi, Acidobacteria, Gemmatimonadetes, Planctomycetes, Verrucomicrobia, Spirochaetes, Fusobacteria, Nitrospirae, Chlorobi, Deferribacteres, Lentisphaerae, Caldiserica, Fibrobacteres, Tenericutes and other 21 candidates (Fig. 2a). The dominant phyla-were Proteobacteria (Alphaproteobacteria, 13.1%; Betaproteobacteria, 13.6%) and Bacteroidetes (30.1 %), which accounted for more than half of the total bacterial sequences (Fig. 2a). The community structure in this study showed a good agreement of soil bacterial communities in other biome (Roesch et al., 2007). However, the bacterial community in this study showed a slightly different community composition compared with other Arctic soil as showing Actinobacteria and Acidobacteria were dominant (Steven et al., 2008; Liebner et al., 2008; Wilhelm et al., 2011).
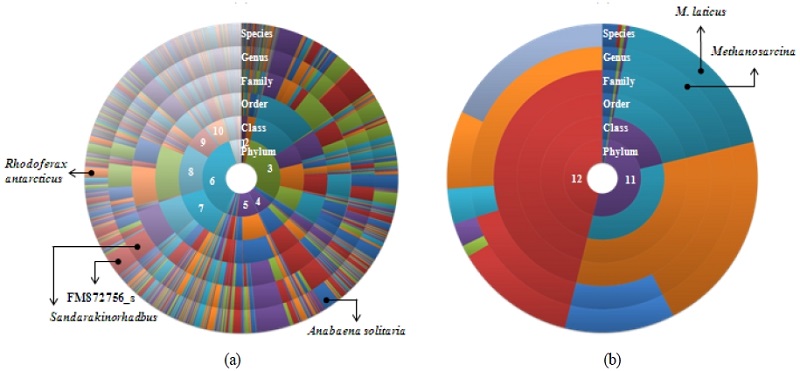
Hierarchical classification of 16S rRNA gene sequences in bacterial (a) and archaeal community (b). The innermost annulus indicates the composition in phylum level; each outer annulus progressively breaks these down by finer taxonomic levels; class, order, genus, and species. 1. Acidobacteria, 2. Actinobacteria, 3. Bacteroidetes,, 4. Cyanobacteria, 5. Firmicutes, 6. Proteobacteria, 7. Alphaproteobacteria, 8. Betaproteobacteria, 9. Deltaproteobacteria, 10. Gammaproteobacteria, 11. Euryarchaeota, 12. Thaumarchaeota.
The difference in dominant bacterial groups may be caused by environmental factors of the sampling site. Acidobacteria and Actinobacteria were dominant bacterial groups in typical soil samples (Chu et al., 2010), and the dominant bands in DGGE analysis of active layer from Canadian high Arctic belonged to Proteobacteria, Bacteroidetes and Actinobacteria (Steven et al., 2008). However, Acidobacteria and Actinobacteria, were a minor group in this study. The sampling site in Resolute was very wet and covered with moss, and the soil pH was relatively higher (pH 7.1∼7.8) (Cruickshank, 1971; Anaka et al., 2008) than usual Arctic soil (pH 4∼5). The pH level of the sampling site was higher than typical Arctic soil which may result in lower number of Acidobacteria in this study. Currently, some studies reported that arctic soil bacterial community composition and diversity are influenced by local variation in soil pH (Chu et al., 2010).
Although a minor portion of reads were classified in species level, relatively dominant species were identified using the 97% similarity. Among the bacterial reads, an OTU was the most dominant sequence with 2.4% in sequence abundance (data not shown). It showed the highest similarity with the sequence FM872756 which was uncultured bacterium isolated from floor dust (Täbel et al., 2009). OTUs showing high sequence similarities with Anabaena solitaria (Cyanobacteria) and Rhodoferax antarcticus (Betaproteobacteria) also predominated with 1.7 % and 1.1% in sequence abundance, respectively. Unfortunately, the understanding of the relationship between community compositions and their potential functions is limited because of the most of OTUs were not cultured.
In case of Archaea, a relatively simple community composition was recognized, detecting Euryarchaeota (51.4%) and Thaumarchaeota (46.1%) as major constituents of the archaeal community (Fig. 2b). Interestingly, the archaeal community composition in this study was significantly different with other active layer soils which showed predominant group was Crenarchaeota (Steven et al., 2008; Wilhelm et al., 2011). Thaumarchaeota which is a newly-proposed phylum has been supposed to play important roles in biogeochemical cycles such as N and C cycles (Muller et al., 2010). The most dominant Archaea were uncultured and poorly provide functional information.
Among archaeal reads, however, an abundant read was affiliated with the Methanosarcina laticus (18.4 %) which might be potentially large source of atmospheric methane (Cadillo-Quiroz et al., 2008). Among the bacterial species, methane oxidizing Proteobacteria belong to Methylococcaceae (0.9%), Methylocystaceae (0.03%) and Beijerinckiaceae (0.01 %) (Bowman et al., 1997; Dedysh et al., 2002; Dedysh et al., 2004) were detected. Therefore, both methane production and consumption processes could occur simultaneously in the active layer soil of Resolute, Canada.
This study showed the composition of bacteria and archaea community in active layer soil of the Canadian high Arctic environment. Proteobacteria and Bacteroidetes were major groups, but Actinobacteria and Acidobacteria was a minor group in Resolute active layer. Euryarchaeota and Thaumarchaeota were dominant in the archaeal community. The microbial community structure showed that methane production and consumption may occur in the active layer soil of Resolute, Canada. Although it might be difficult to characterize microbial community with only one sample in this habitat, it could be used for the basis of assessing the relative importance of the specific groups with a high resolution on the shaping of the bacterial and archaeal community in Arctic active layer.
Acknowledgments
We thank Dr. Jee, Geonhwa for his supporting the sampling. This study was supported by a grant from the National Research Foundation of Korea funded by the Korean Government (MSIP) (NRF-2011-0021067) (PN14082, KOPRI), and PK09100 from Korea Polar Research Institute (KOPRI).
References
-
Anaka , A, Wickstrom , M, Siciliano , SD, Biogeochemical toxicity and phytotoxicity of nitrogenous compounds in a variety of arctic soils, Environmental Toxicology and Chemistry, (2008), 27, p1809-1816.
[https://doi.org/10.1897/07-567.1]
-
Bowman , JP, McCammon , SA, Skerratt , JH, Methylosphaera hansonii gen. nov., sp. nov., a psychrophilic, group I methanotroph from Antarctic marine-salinity, meromictic lakes, Microbiol, (1997), 143, p 1451-1459.
[https://doi.org/10.1099/00221287-143-4-1451]
-
Cadillo-Quiroz, H, Yashiro , E, Yavitt , JB, Zinder , SH, Characterization of the archaeal community in a minerotrophic fen and terminal restriction fragment length polymorphism-directed isolation of a novel hydrogenotrophic methanogen, Appl Environ Microbiol, (2008), 74, p2059-2068.
[https://doi.org/10.1128/AEM.02222-07]
-
Christensen , TR, Johansson , T, Ǻkerman , HJ, Mastepanov , M, Malmer , N, Friborg , T, Crill , P, Svensson , BH, Thawing sub-arctic permafrost: effects on vegetation and methane emissions, Geophysical Research Letters, (2004), 31, pL04501.
[https://doi.org/10.1029/2003GL018680]
-
Chu , H, Fierer , N, Lauber , CL, Caporaso , JG, Knight , R, Grogan , P, Soil bacterial diversity in the Arctic is not fundamentally different from that found in other biomes, Environ Microbiol, (2010), 12, p 2998-3006.
[https://doi.org/10.1111/j.1462-2920.2010.02277.x]
-
Cruickshank , JG, Soils and Terrain Units around Resolute, Cornwallis Island, Arctic, (1971), 24, p195-209.
[https://doi.org/10.14430/arctic3133]
- Dedysh , SN, Khmelenina , VN, Suzina , NE, Trotsenko , YA, Semrau , JD, Liesack , W, Tiedje , JM, Methylocapsa acidiphila gen. nov., sp. nov., a novel methane-oxidizing and dinitrogen-fixing acidophilic bacterium from Sphagnum bog, Int J Syst Evol Microbiol, (2002), 52, p251-261.
-
Dedysh , SN, Berestovskaya , YY, Vasilieva , LV, Belova , SE, Khmelenina , VN, Suzina , NE, Trotsenko , YA, Liesack , W, Zavarzin , GA, Methylocella tundrae sp. nov., a novel methanotrophic bacterium from acidic tundra peatlands, Int J Syst Evol Microbiol, (2004), 54, p151-156.
[https://doi.org/10.1099/ijs.0.02805-0]
-
DeLong , EF, Archaea in coastal marine environments, Proc Natl Acad Sci USA, (1992), 89, p5685-5689.
[https://doi.org/10.1073/pnas.89.12.5685]
- Environment Canada, Canadian climate normals. http://climate.weather.gc.ca/, (2014).
- Guglielmin , M, Observations on permafrost ground thermal regimes from Antarctica and the Italian Alps, and their relevance to global climate change, Global and Planetary Change, (2004), 40, p159-167.
-
Hansel , CM, Fendorf , S, Jardine , PM, Francis , CA, Changes in bacterial and archaeal community structure and functional diversity along a geochemically variable soil profile, Appl Environ Microbiol, (2008), 74, p1620-1633.
[https://doi.org/10.1128/AEM.01787-07]
-
Hughes , JB, Hellmann , JJ, Ricketts , TH, Bohannan , BJM, Counting the uncountable: statistical approaches to estimating microbial diversity, Appl Environ Microbiol, (2001), 67, p4399-4406.
[https://doi.org/10.1128/AEM.67.10.4399-4406.2001]
-
Kim , OS, Cho , YJ, Lee , K, Yoon , SH, Kim , M, Na , H, Park , SC, Jeon , YS, Lee , JH, Yi , H, Won , S, Chun , J, Introducing EzTaxon-e: a prokaryotic 16S rRNA Gene sequence database with phylotypes that represent uncultured species, Int J Syst Evol Microbiol, (2012), 62, p716-721.
[https://doi.org/10.1099/ijs.0.038075-0]
-
Lawrence , DM, Slater , AG, A projection of severe near-surface permafrost degradation during the 21st century, Geophysical Research Letters, (2005), 32, pL24401.
[https://doi.org/10.1029/2005GL025080]
-
Li , W, Godzik , A, Cd-hit: a fast program for clustering and comparing large sets of protein or nucleotide sequences, Bioinformatics, (2006), 22, p1658-1659.
[https://doi.org/10.1093/bioinformatics/btl158]
-
Liebner , S, Rublack, Stuehrmann , T, Wagner , D, Diversity of aerobic methanotrophic bacteria in a permafrost active layer soil of the Lena Delta, Siberia, Microb Ecol, (2009), 57, p25-35.
[https://doi.org/10.1007/s00248-008-9411-x]
- Liebner , S, Harder , J, Wagner , D, Bacterial diversity and community structure in polygonal tundra soils from Samoylov Island, Lena Delta, Siberia, Int Microbiol, (2008), 11, p195-202.
-
Martineau , C, Whyte , LG, Greer , CW, Stable isotope probing analysis of the diversity and activity of methanotrophic bacteria in soils from the Canadian high Arctic, Appl Environ Microbiol, (2010), 76, p 5773-5784.
[https://doi.org/10.1128/AEM.03094-09]
-
Muller , F, Brissac , T, Le Bris, N, Felbeck , H, Gros , O, First description of giant Archaea (Thaumarchaeota) associated with putative bacterial ectosymbionts in a sulfidic marine habitat, Environ Microbiol, (2010), 12, p2371-2383.
[https://doi.org/10.1111/j.1462-2920.2010.02309.x]
-
Roesch , LFW, Fulthorpe , RR, Riva , A, Casella , G, Hadwin , AK, Kent , AD, Daroub , SH, Camargo , FA, Farmerie , WG, Triplett , EW, Pyrosequencing enumerates and contrasts soil microbial diversity, ISME J, (2007), 1, p283-290.
[https://doi.org/10.1038/ismej.2007.53]
-
Ronaghi , M, Pyrosequencing sheds light on DNA sequencing, Genome Res, (2001), 11, p3-11.
[https://doi.org/10.1101/gr.11.1.3]
-
Schloss , PD, Westcott , SL, Ryabin , T, Hall , JR, Hartmann , M, Hollister , EB, Lesniewski , RA, Oakley , BB, Parks , DH, Robinson , CJ, Sahl , JW, Stres , B, Thallinger , GG, Van Horn , DJ, Weber , CF, Introducing mothur: open-source, platform independent, community-supported software for describing and comparing microbial communities, Appl Environ Microbiol, (2009), 75, p7537-7541.
[https://doi.org/10.1128/AEM.01541-09]
- Stackebrandt , E, Goebel , B, Taxonomic note: a place for DNA-DNA reassociation and 16S r-RNA sequence analysis in the present species definition in bacteriology, Int J Syst Bacteriol, (1994), 44, p 846-849.
-
Steven , B, Pollard , WH, Greer , CW, Whyte , LG, Microbial diversity and activity through a permafrost/ ground ice core profile from the Canadian high Arctic, Environ Microbiol, (2008), 10, p3388-3403.
[https://doi.org/10.1111/j.1462-2920.2008.01746.x]
-
Qian , PY, Wang , Y, Lee , OO, Lau , SCK, Yang , JK, Lafi , FF, Al-Suwailem, A, Wong , TYH, Vertical stratification of microbial communities in the Red sea revealed by 16S rDNA pyrosequencing, ISME J, (2011), 5, p507-518.
[https://doi.org/10.1038/ismej.2010.112]
- Täbel , M, Rintala , H, Pitkaranta , M, Paulin , L, Kaitinen , S, Pekkanen , J, Hyvrinen , A, Nevalainen , A, The occupant as a source of house dust bacteria, J Allergy Clin Immunol, (2009), 124, p834-840.
- Weisburg , WG, Barns , SM, Pelletier , DA, Lane , DJ, 16S ribosomal DNA amplification for phylogenetic study, J Bacteriol, (1991), 173, p697-703.
-
Wilhelm , RC, Niederberger , TD, Greer , C, Whyte , LG, Microbial diversity of active layer and permafrost in an acidic wetland from the Canadian High Arctic, Can J Microbiol, (2011), 57, p303-315.
[https://doi.org/10.1139/w11-004]
-
Yergeau , E, Hogues , H, Whyte , LG, Greer , CW, The functional potential of high Arctic permafrost revealed by metagenomic sequencing, qPCR and microarray analyses, ISME J, (2010), 4, p1206-1214.
[https://doi.org/10.1038/ismej.2010.41]
-
Zhou , J, Davey , ME, Figueras , JB, Rivkina , E, Gilichinsky , D, Tiedje , JM, Phylogenetic diversity of a bacterial community determined from Siberian tundra soil DNA, Microbiology, (1997), 143, p3913-3919.
[https://doi.org/10.1099/00221287-143-12-3913]
- Zimov , SA, Schuur , EAG, Chapin , FS, Permafrost and the global carbon budget, Science, (2007), 312, p1612-1613.