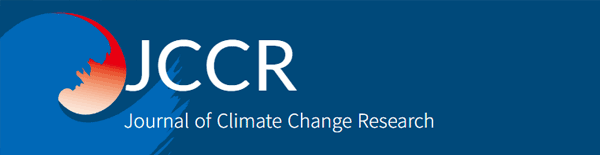
실외 실험적 온난화 및 강수 조절 하에서 토양 수분과 소나무 묘목의 생리 및 생장 반응 간의 관계
Abstract
We investigated the changes in soil water and physiological and growth responses of Pinus densiflora seedlings under open‐field experimental warming and precipitation manipulation. In April 2013, we planted 2‐year‐old P. densiflora seedlings at 2 temperature levels (control, +3°C warmed) × 3 precipitation levels (control, ‐30% decreased, +30% increased) × 3 replicates (n=18). Soil water content (SWC), net photosynthetic rate (Pn), stomatal conductance (gs), transpiration rate (E), intrinsic water use efficiency (iWUE), and total chlorophyll content (Tchl.) were measured in April, May, July, and August 2015, and the growth rate was calculated for April 2015‐March 2016. SWC significantly decreased by warming and precipitation manipulation. The rate of change in SWC was 12.17% under warming, but 5.13% and 6.53% under decreased and increased precipitation, respectively. Pn, gs, and E decreased significantly by 17.09%, 18.25%, and 16.76% under warming, while only E changed by ‐5.29% and 20.28% under decreased and increased precipitation, respectively. There were no significant changes in iWUE or Tchl. by warming or precipitation manipulation. SWC was correlated with Pn, gs, and E in April and May, and Pn in July, but was not correlated with any physiological properties in August. Seedling growth rates of root collar diameter and height were not changed despite the decrease in Pn related to warming. In this study, the effect of warming on physiological responses was greater than that of precipitation manipulation, and Pn decreased due to stomatal closure induced by soil water reduction under warming. These responses changed with seasonal environmental changes.
Keywords:
Climate Change, Growth, Physiology, Pinus densiflora, Soil Water Content1. 서 론
IPCC 보고서에 의하면 기후변화에 따라 전 지구적으로 1850-1900년에 비하여 2100년대에 기온이 1.5°C 이상 증가할 것으로 보이며, 지역에 따라 강수량 및 그 유형이 변화할 것으로 예상되고 있다 (IPCC, 2013). 수목의 생리 및 생장은 온도와 강수 변화에 민감하게 반응하며, 기후변화에 따른 수목의 생리 및 생장의 변화는 산림 생태계의 동태와 탄소 순환에 영향을 준다 (Walther et al., 2002).
한편, 온도 증가와 가뭄으로 온대 침엽수림에서 수목의 고사가 증가하고 있으며 (Allen et al., 2010), 국내에서도 기후변화의 영향으로 침엽수의 고사 현상이 나타나고 있다 (Lim and Shin, 2005). 이는 기후변화가 수목에 열 및 수분 스트레스 등을 발생시켰기 때문으로 보고되었다 (Prieto et al., 2009; Sevanto et al., 2014).
그러나 기존 연구에 의하면 온난화 및 강수량 변화에 대한 수목의 반응은 다양하게 나타났다 (Allen et al., 2010; Sevanto et al., 2014). 침엽수를 대상으로 한 실험적 기후변화 연구에서 잎의 광합성은 온도 및 강수 조절에 따라 증가하거나 (Herguido et al., 2016; Tang et al., 2016), 감소하였다 (Herguido et al., 2016; Matías et al., 2017; Prieto et al., 2009). 이는 수종별로 열 및 수분 스트레스에 대응하기 위하여 기공전도도가 광합성을 조절하는 기작이 다르기 때문인 것으로 보인다 (Allen et al., 2010; Sevanto et al., 2014). 등수성 수종은 수분 변화에 빠르게 반응하며 토양 수분이 감소하면 기공을 폐쇄하고, 비등수성 수종은 환경변화에 따라 기공을 조절하지 않고 광합성을 유지하는 경향을 보인다 (Garcia‐Forner et al., 2016). 또한 광합성의 변화와 마찬가지로 침엽수의 생장도 온도 및 강수량 변화에 의해 증가하거나 (Yin et al., 2008; Zhao and Liu, 2008) 감소하는 것으로 보고된 바 있다 (Matías et al., 2017).
RCP 8.5 시나리오에 따르면 50년 후 한국의 기온은 3°C 증가하며 강수량은 30% 증가할 것으로 예측된다 (Korea Meteorological Administration, 2018). 그리고 우리나라의 대표적인 침엽수종인 소나무 역시 온도 및 강수량 변화에 의한 스트레스로 산림 면적과 생장이 감소할 것으로 예상된다 (Byun et al., 2010). 따라서 본 연구에서는 실외 실험적 온난화 및 강수 조절 시스템을 조성하고 기후변화에 따른 토양 환경과 소나무 묘목의 생리 및 생장 반응 및 그 관계를 구명하고자 하였다. 특히, 묘목 단계는 환경의 변화에 민감하게 반응하며, 이후의 수목의 생장에 큰 영향을 주기 때문에 기후변화에 따른 묘목의 반응을 파악하는 것은 중요하다 (Niinemets, 2010).
본 연구에서는 다음과 같은 가설을 설정하였다. (1) 온난화 및 강수 감소에 따라 토양 수분이 감소하며, 강수 증가에 따라 토양 수분이 증가할 것이다. (2) 등수성 수종인 소나무 묘목은 토양 수분의 변화에 따라 기공전도도를 감소 또는 증가시킬 것이다. (3) 잎의 기공전도도는 광합성 및 묘목의 생장과 양의 상관관계를 보일 것이다.
2. 재료 및 방법
2.1 연구 설계
본 연구는 고려대학교 환경생태수목원 실외 묘포장에서 수행되었으며 (37° 35′36″N, 127° 1′31″E), 이 곳의 2015년 연평균온도 및 연강수량은 각각 13.3°C와 792.1 mm이었다. 연구대상지의 토양은 양질사토 (모래 80%, 미사 14%, 점토 6%)이며, pH 6.52, 총탄소농도 0.22%, 총질소농도 0.05%, 양이온치환용량 3.67 cmolc kg-1이다 (Yun et al., 2014). 2013년 4월, 연구지에 18개의 실험구 (1.5 m × 1.5 m)를 조성하고 각 실험구마다 2년생 소나무 묘목을 각각 45본씩 식재하였고, 2014년 4월에 종묘사업실시요령 (20본 m-2)에 따라 묘목을 재이식하였다. 실험구는 2개 온도 조절 (온도대조 (C), 온난화 (W)) × 3개 강수 조절 (강수대조 (P0), 강수감소 (P-), 강수증가 (P+)) × 3개 반복으로 구성하였다. 적외선등을 평균 묘고로부터 80 cm 높이에 설치하고 데이터로거 및 릴레이를 이용하여 온난화구의 대기온도가 온도대조구보다 3°C 높게 유지되도록 하였다. 강수감소구에는 지면으로부터 평균 160 cm 높이에 실험구 면적의 30%를 덮도록 V자형의 광투과성 패널을 설치하여 강수를 차단하였다. 차단된 강수는 파이프를 통하여 물탱크에 모이고 일정량 이상이 되면 강수증가구에 점적관수하였다. 구조물에 의한 환경 변화를 줄이기 위하여 온도대조구에는 적외선등 모형을 설치하였으며, 강수대조구 및 강수증가구에는 강수를 차단하지 않는 일자형 광투과성 패널을 설치하였다 (Yun et al., 2014). 한편 적설로 인한 붕괴 위험을 방지하고자 매년 1월과 2월에는 광투과성 패널을 제거하였다. 각 실험구에 토양 수분 센서 (CS616, Campbell Scientific Inc., USA)를 한 개씩 설치하여 토양 수분 함량을 측정하고 2015년 4월부터 8월의 월 평균 토양 수분 함량 값을 산출하였다.
2.2 잎의 생리 및 생장 반응 측정
잎의 가스교환은 2015년 4월, 5월, 7월, 8월의 상순에 실험구 당 임의의 묘목 3본을 선정하고 묘목 당 6개의 생장이 완료된 잎을 채취한 후 맑은 날 오전에 CIRAS2 (PP Systems, USA)로 측정하였다. 측정 시 챔버 (80 mm × 30 mm) 내 CO2 농도는 400 ppm, 광합성광양자밀도 (PPFD)는 1,000–1,100 μmol m-2 s-1로 유지하였다. 가스 교환 측정에 이용한 잎의 엽면적은 Perfection 4990 scanner (EPSON, Japan) 및 WinSEEDLE (Regent, Canada) 프로그램으로 측정하였으며, 이를 이용하여 측정한 가스교환 값을 환산하여 단위 면적 당 순광합성률 (Pn; μmol CO2 m-2 s-1), 기공전도도 (gs; mmol H2O m-2 s-1) 및 증산속도 (E; mmol H2O m-2 s-1)를 계산하였다. 내재적 수분이용효율 (iWUE; μmol CO2 mmol-1 H2O)은 잎의 순광합성률을 기공전도도로 나누어 산출하였다. 또한 채취한 잎에서 dimethyl sulfoxide (DMSO) 용액으로 엽록소를 추출하고 분광광도계 (U-1100, Hitachi, Japan)로 665 nm 및 648 nm 파장에서 추출액의 흡광도를 측정하여 총엽록소 함량을 산출하였다 (Barnes et al., 1992).
묘목의 근원경 및 묘고는 2015년 4월과 2016년 3월에 디지털 캘리퍼와 절척을 이용하여 측정하였다. 근원경은 지면으로부터 1 cm 위에 표시하고 같은 위치에서 반복 측정하였으며, 묘고는 지면으로부터 정아까지의 길이를 측정하였다. 그리고 2015년 4월부터 2016년 3월까지의 생장량을 2015년 4월에 측정한 값으로 나누어 생장률을 산출하였다.
2.3 통계 분석
온난화, 강수 조절 및 측정 시기가 토양 수분 함량, 잎의 순광합성률, 기공전도도, 증산속도, 내재적 수분이용효율, 총엽록소 함량 등에 미치는 영향은 PROC GLIMMIX 절차를 이용한 반복측정분산분석으로 검정하였으며 Tukey 사후 검정을 수행하였다. 측정 시기 (월)별 토양 수분 함량과 생리적 특성간의 관계는 상관분석을 통하여 검정하였다. 온난화 및 강수 조절이 묘목의 근원경 및 묘고 생장률에 미치는 영향은 이원분산분석으로 검정하였으며 Tukey 사후 검정을 수행하였다. 모든 분석은 SAS 9.4 (SAS Institute Inc., USA)를 이용하였으며, 유의수준은 p<0.05로 하였다.
3. 결과
토양 수분 함량은 온난화, 강수 조절 및 측정 시기에 따라 유의한 차이를 보였으나, 온난화 및 강수 조절의 교호작용에 의한 유의한 차이는 없었다 (Table 1; Figure 1). 실험구별 평균 토양 수분 함량 (vol %)은 CP0: 6.77±0.33, CP—: 6.32±0.15, CP+: 7.15±0.17, WP0: 5.85±0.45, WP—: 5.64±0.08, WP+: 6.29±0.20 등으로, 온난화에 의하여 12.17% 감소하였고 (p=0.0001), 강수 감소에 의하여 5.13% 감소하였으며, 강수 증가에 의하여 6.53% 증가하였다 (p=0.026). 강수 조절에 따른 토양 수분 함량 차이의 사후 검정 결과에 따르면 P0 실험구와 P— 또는 P+ 실험구 간의 유의한 차이는 없으며 P—와 P+ 실험구 간에만 유의한 차이를 보였다.

Results of GLIMMIX analysis for soil environments and physiological responses of Pinus densiflora seedlings
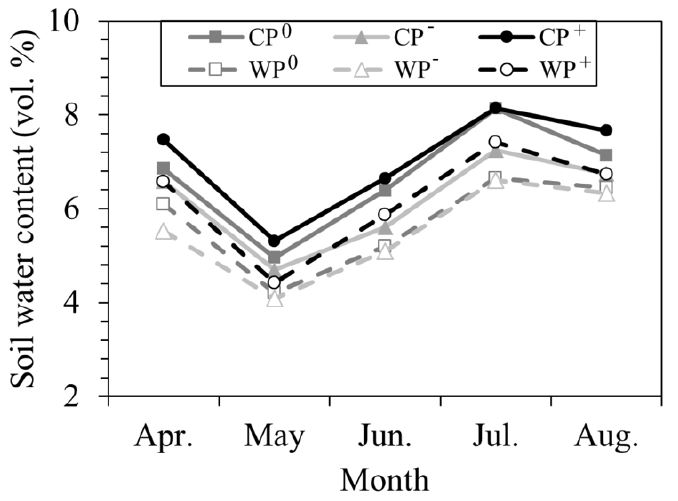
Soil water content from April to August 2015 under experimental warming and precipitation manipulation. CP0: control temperature and control precipitation, CP—: control temperature and decreased precipitation, CP+: control temperature and increased precipitation, WP0: warmed temperature and control precipitation, WP—: warmed temperature and decreased precipitation, WP+: warmed temperature and increased precipitation.
소나무 묘목 잎의 기공전도도 (mmol H2O m-2 s-1)는 온난화 및 측정 시기에 따라 유의한 차이를 보였다 (Table 1). 실험구별 평균 기공전도도는 CP0: 159.30±20.82, CP—: 153.93±19.43, CP+: 174.02±15.21, WP0: 122.49±11.79, WP—: 116.19±18.87, WP+: 161.60±11.16 등으로 온난화에 의한 변화율은 P0, P—, P+ 실험구에서 각각 -23.10%, -24.52%, -7.14%로 나타났다 (Figure 2a). 온난화에 따른 월별 기공전도도의 변화율은 4월, 5월, 7월, 8월에 각각 -13.62%, -39.17%, -16.65%, -8.01%로 나타났다 (Figure 2a).
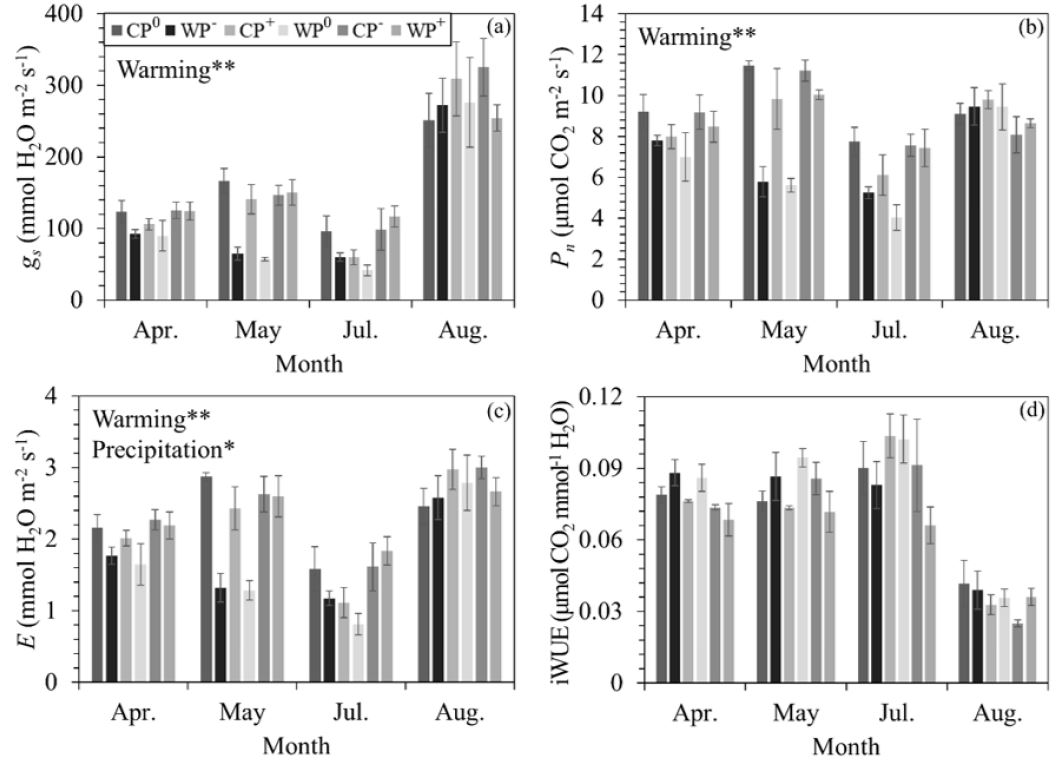
Stomatal conductance (gs,a), net photosynthetic rate (Pn,b), transpiration (E,c), intrinsic water use efficiency (iWUE, d) under warming and precipitation manipulation. Abbreviations of the plots are as in Figure 1. Error bars are the standard errors of the means, and asterisk indicates significant difference by treatments (*p<0.05, **p<0.01).
순광합성률 (μmol CO2 m-2 s-1)은 온난화 및 측정 시기에 따라 유의한 차이를 보였다 (Table 1). 실험구별 평균 순광합성률은 CP0: 9.38±0.49, CP—: 8.43±0.71, CP+: 9.01±0.44, WP0: 7.08±0.42, WP—: 6.52±0.45, WP+: 8.65±0.07 등으로 온난화에 의한 변화율은 P0, P—, P+ 실험구에서 각각 -24.57%, -22.67%, -4.05%로 나타났다 (Figure 2b). 온난화에 따른 월별 순광합성률의 변화율은 4월, 5월, 7월, 8월에 각각 -11.85%, -34.28%, -22.65%, 2.41%로 나타났다 (Figure 2b).
증산속도 (mmol H2O m-2 s-1)는 온난화, 강수 조절, 측정 시기에 따라 유의한 차이를 보였고 (Table 1), 실험구별 평균 증산속도는 CP0: 2.27±0.17, CP—: 2.31±0.20, CP+: 2.38±0.12, WP0: 1.71±0.12, WP—: 1.63±0.10, WP+: 2.32±0.16 등이었다. 온난화에 의한 증산속도의 변화율은 P0, P—, P+ 실험구에서 각각 -24.50%, -23.43%, -2.36%로 나타났다 (Figure 2c). 온난화에 따른 월별 순광합성률의 변화율은 4월, 5월, 7월, 8월에 각각 -13.21%, -34.08%, -13.02%, -4.18% 등으로 나타났다 (Figure 2c). 강수 조절에 따른 증산속도의 변화율은 강수 감소에 따라 -5.29%, 강수 증가에 따라 20.28%로 나타났으며, 사후 검정 결과에 따르면 P—와 P+ 실험구 간에만 유의한 차이를 보였으며 대조구와 처리구 간의 유의한 차이는 나타나지 않았다 (Figure 2c).
잎의 내재적 수분이용효율은 측정 시기에 의해서만 유의한 차이를 보였으며, 온도 및 강수 조절 처리에 의한 영향은 나타나지 않았다 (CP0: 0.07±0.01, CP—: 0.07±0.00, CP+: 0.07±0.00, WP0: 0.07±0.01, WP—: 0.08±0.00, WP+: 0.06±0.01; Table 1, Figure 2d).
한편 온난화 및 강수 조절 처리의 교호작용의 영향은 모든 생리적 특성에서 나타나지 않았다 (Table 1).
실험구별 평균 총엽록소 함량은 CP0: 1.27±0.01, CP—: 1.42±0.04, CP+: 1.31±0.07, WP0: 1.27±0.07, WP—: 1.41±0.06, WP+: 1.26±0.10 등으로 측정 시기에 따라서만 유의한 차이를 보였으며 온난화 및 강수 조절에 의한 차이는 나타나지 않았다 (Figure 3, Table 1).
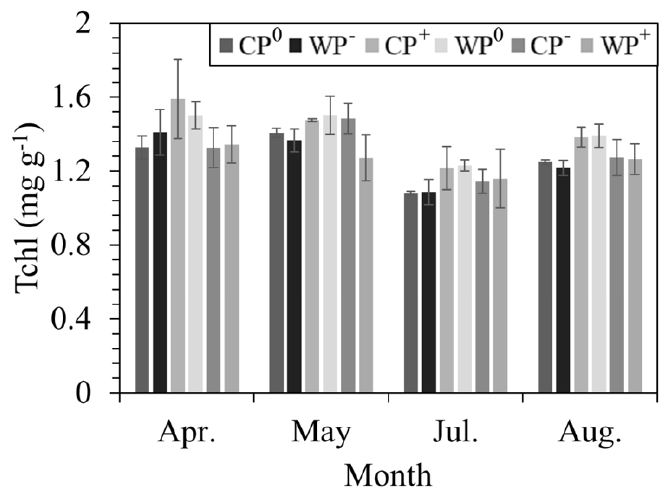
Total chlorophyll content (Tchl) under warming and precipitation manipulation. Abbreviations of the plots are as in Figure 1. Error bars are the standard errors of the means.
토양 수분 함량과 내재적 수분이용효율 및 총엽록소 함량 간의 상관관계는 유의하지 않았으며 토양 수분 함량과 가스교환 (기공전도도, 순광합성률 및 증산속도) 간의 상관관계는 월별로 다르게 나타났다 (Table 2). 4월과 5월에는 토양 수분 함량과 모든 가스교환 간 유의한 양의 상관관계를 보였으며, 7월에는 순광합성률만 토양 수분 함량과 유의한 양의 상관관계를 보였다 (r=0.60 p=0.008). 그리고 8월에는 토양 수분 함량과 모든 생리적 특성 간의 유의한 상관관계가 나타나지 않았다.

Correlation coefficient of physiological responses of Pinus densiflora seedlings with soil water content
묘목의 근원경 및 묘고의 생장률은 각각 34.15-45.38%와 32.08-46.25%의 범위로 나타났으며, 온난화, 강수 조절 및 두 처리 간 교호작용의 영향은 나타나지 않았다 (Table 3, 4).

Results of two-way ANOVA for growth rate of root collar diameter (RCD) and height from April 2015 to March 2016
4. 고찰
기후변화가 진행됨에 따라 강수량의 변화뿐 아니라 온도의 증가도 토양 수분에 영향을 미치는 주요인으로 보고된 바 있다 (Dai, 2011; Han et al., 2017). 본 연구에서 토양 수분 함량은 온난화 및 강수 조절에 따라 유의한 차이를 보였는데 온난화에 의한 토양 수분 함량의 변화율이 더 큰 것으로 나타났다. 또한 강수 대조구와 비교하여 강수 조절에 따른 토양 수분 함량의 변화는 유의하지 않았다. 2015년 서울의 연강수량은 792.1 mm이었으며, 이는 1981년부터 2010년까지 서울의 평년강수량 (1450.5 mm)의 54.6%에 불과하다 (Korea Meteorological Administration, 2015). 2015년에는 절대적인 강수량이 적기 때문에 강수 조절에 따른 토양 수분 함량의 변화가 크지 않은 것으로 판단된다.
본 연구에서 소나무 묘목의 기공전도도, 순광합성률, 증산속도는 온난화에 의하여 감소하는 경향을 보였다. 또한 4, 5월의 기공전도도와 증산속도 및 4, 5, 7월의 순광합성률은 토양 수분 함량과 양의 상관관계를 보였다. 이는 가설과 같이 토양 수분이 감소하면 기공을 닫아 잎의 수분을 유지하는 등수성 수종인 소나무류의 특성에 의한 것으로 보인다 (Sala et al., 2010). 또한 기공 폐쇄로 인하여 순광합성률 및 증산속도도 감소한 것으로 판단된다 (Prieto et al., 2009). 기공전도도와 함께 순광합성률이 감소하였으므로 수분이용효율은 온난화 처리에도 변화하지 않은 것으로 사료된다. 또한 총엽록소 함량은 잎의 수분포텐셜이 감소할 경우 감소한다고 보고되었으나 (Guerfel et al., 2009), 본 연구에서 소나무 묘목은 토양 수분의 감소에 따라 기공전도도를 감소시켜 잎의 수분 함량을 유지하였으므로 총엽록소 함량에는 변화가 없었던 것으로 보인다.
본 연구에서 강수 조절은 증산속도를 제외한 생리적 특성에 영향을 주지 못한 것으로 나타났다. 강수 조절에 따른 증산속도의 차이는 강수 증가와 강수 감소 처리 간에만 유의하였으며 이는 강수 조절에 따른 토양 수분 함량의 반응과 같은 경향을 보였다. 강수 조절 하에서 토양 수분 함량의 변화가 적어 이에 따른 생리적 특성의 반응도 변화율이 낮은 것으로 판단된다. 한편, 다른 강수 조절과 비교하여 강수 증가 처리 하에서는 온난화에 의하여 기공전도도, 순광합성률, 증산속도가 감소하는 비율이 낮게 나타났다. 즉, 강수 증가 처리가 온난화에 따른 생리적 특성의 감소 효과를 상쇄시킨 것으로 생각된다 (Reich et al., 2018).
한편, 온난화 및 강수 조절에 따른 8월의 생리적 반응은 4, 5, 7월과 다른 경향을 보였다. 다른 월에 비하여 8월의 기공전도도는 2.7배 증가하였으며 수분이용효율은 2.4배 감소하였다. 그리고 증산속도도도 8월에 가장 높은 값을 보였다. 또한 토양 수분과 모든 생리적 특성 간의 상관관계가 유의하지 않았다. 이는 수분이 충분한 조건에서 고온으로 인하여 높아진 잎의 온도를 낮추기 위하여 기공을 개방하고 증산속도를 증가시켰기 때문으로 보인다 (Crawford et al., 2012). 2015년 7월 (25.8ºC)과 8월 (26.3ºC)의 기온은 유사하게 나타난 반면 서울의 강수량은 6월 20일부터 7월 10일까지 18 mm, 7월 20일부터 8월 10일까지 215.6 mm로 나타났다 (Korea Meteorological Administration, 2015). 8월 상순에 생리적 반응을 측정하기 전 많은 강수량으로 인하여 토양에 충분한 수분이 공급되었고 이에 따라 유사한 기온의 7월과는 다른 경향이 나타난 것으로 보인다.
등수성 수종은 지속적으로 건조한 환경에 노출되면 기공 폐쇄에 따른 탄소 기아의 위험이 있다 (Allen et al., 2010). 본 연구에서 온난화에 의하여 순광합성률이 감소하였으며, 같은 연구 시스템에서 2015년 이전에 수행된 연구에서도 소나무 묘목은 온난화 처리에 따라 기공전도도 및 순광합성률이 감소하는 현상을 보였다 (Han et al., 2018, Yun et al., 2016). 그러나 소나무 묘목의 생장률은 변화하지 않았다. 이는 환경에 대한 생장 반응은 생리적 특성의 변화 이후 장기적으로 서서히 변화하기 때문으로 보인다 (Chapin and Shaver, 1996).
5. 결론
본 연구 결과에 따르면 온난화 및 강수 조절에 따라 토양 수분이 변화하고 이에 따라 소나무 묘목의 생리적 특성이 영향을 받는 것으로 나타났는데, 연구기간 동안 낮은 강수량으로 온난화의 영향이 강수 조절의 영향보다 큰 것으로 나타났다. 등수성 수종인 소나무 묘목은 온난화에 따른 토양 수분 감소에 따라 기공 폐쇄하고 생리적 활성이 감소하였다. 강수 조절은 증산 속도에만 영향을 미치는 것으로 나타났으며, 강수 증가는 온난화에 의한 생리적 활성 감소 효과를 일부 상쇄시키는 것으로 보인다. 한편, 소나무 묘목의 생리적 반응은 계절의 변화에 따라 8월의 온도 및 강수량이 증가한 조건에서는 온난화 및 강수 조절의 영향이 나타나지 않았으며 4월부터 7월초까지 건조한 기간에 감소하는 것으로 나타났다. 본 연구 결과를 활용하여 봄철 건조한 기간에 토양 수분 모니터링을 통한 묘목 관리를 할 수 있을 것으로 생각된다. 그러나 이는 단기간에 수행된 연구로 묘목의 생리 및 생장 반응에 대한 장기적인 기후변화 영향의 분석이 요구된다.
Acknowledgments
본 연구는 연구재단 (NRF-2013R1A1A2012242) 및 산림청 (2017058A00-1919-AB01)의 지원으로 수행되었습니다.
References
-
Allen, CD, Macalady, AK, Chenchouni, H, Bachelet, D, McDowell, N, Vennetier, M, Kitzberger, T, Rigling, A, Breshears, DD, Hogg, EH, Gonzalez, P, Fensham, R, Zhangm, Z, Castro, J, Demidova, N, Lim, JH, Allard, G, Running, SW, Semerci, A, Cobb, N., (2010), A global overview of drought and heat-induced tree mortality reveals emerging climate change risks for forests, For Ecol Manag, 259, p660-684.
[https://doi.org/10.1016/j.foreco.2009.09.001]
-
Barnes, JD, Balaguer, L, Manrique, E, Elvira, S, Davison, AW., (1992), A reappraisal of the use of DMSO for the extraction and determination of chlorophylls a and b in lichens and higher plants, Environ Exp Bot, 32, p85-100.
[https://doi.org/10.1016/0098-8472(92)90034-y]
- Byun, JG, Lee, WK, Nor, DK, Kim, SH, Choi, JK, Lee, YJ., (2010), The relationship between tree radial growth and topographic and climatic factors in red pine and oak in central regions of Korea, J Korean For Soc, 99, p908-913, (In Korean with English abstract).
-
Chapin, FS, Shaver, GR., (1996), Physiological and growth responses of arctic plants to a field experiment simulating climatic change, Ecology, 77, p822-840.
[https://doi.org/10.2307/2265504]
-
Crawford, AJ, McLachlan, DH, Hetherington, AM, Franklin, KA., (2012), High temperature exposure increases plant cooling capacity, Curr Biol, 22, p396-397.
[https://doi.org/10.1016/j.cub.2012.03.044]
-
Dai, A., (2011), Drought under global warming: a review, WIREs Clim Change, 2, p45-65.
[https://doi.org/10.1002/wcc.81]
- Garcia‐Forner, N, Adams, HD, Sevanto, S, Collins, AD, Dickman, LT, Hudson, PJ, Zeppel, MJB, Jenkins, MW, Powers, H, Martínez-Vilalta, J, Mcdowell, NG., (2016), Responses of two semiarid conifer tree species to reduced precipitation and warming reveal new perspectives for stomatal regulation, Plant Cell Environ, 39, p38-49.
-
Guerfel, M, Baccouri, O, Boujnah, D, Chaïbi, W, Zarrouk, M., (2009), Impacts of water stress on gas exchange, water relations, chlorophyll content and leaf structure in the two main Tunisian olive (Olea europaea L.) cultivars, Sci Hortic, 119, p257-263.
[https://doi.org/10.1016/j.scienta.2008.08.006]
-
Han, SH, Kim, S, Li, G, Chang, H, Yun, SJ, An, J, Son, Y., (2017), Effects of warming and precipitation manipulation on fine root dynamics of Pinus densiflora Sieb. et Zucc. seedlings, Forests, 9, p14.
[https://doi.org/10.3390/f9010014]
-
Herguido, E, Granda, E, Benavides, R, García-Cervigón, AI, Camarero, JJ, Valladares, F., (2016), Contrasting growth and mortality responses to climate warming of two pine species in a continental Mediterranean ecosystem, For Ecol Manag, 363, p149-158.
[https://doi.org/10.1016/j.foreco.2015.12.038]
- Intergovernmental Panel on Climate Change (IPCC), (2013), Summary for policymakers, In Stocker, T, (ed)Climate change 2013: The physical science basis, Working group I contribution to the fifth assessment report of the intergovernmental panel on climate change New York, Cambridge University Press, p33-118.
- Korea Meteorological Administration, (2015), Annual climatological report, Seoul, Korea Meteorological Administration.
- Korea Meteorological Administration, (2018), Climate Information Portal, [accessed 2018 Apr 1], http://www.climate.go.kr/personal_RCP/new/korea_step1.php.
- Lim, JH, Shin, JH., (2005), Forest vegetation shift and plant phenological changes according to global warming, Nat Conserv, 130, p8-17, (In Korean).
-
Matías, L, Castro, J, Villar-Salvador, P, Quero, JL, Jump, AS., (2017), Differential impact of hotter drought on seedling performance of five ecologically distinct pine species, Plant Ecol, 218, p201-212.
[https://doi.org/10.1007/s11258-016-0677-7]
-
Niinemets, U., (2010), Responses of forest trees to single and multiple environmental stresses from seedlings to mature plants: past stress history, stress interactions, tolerance and acclimation, For Ecol Manag, 260, p1623-1639.
[https://doi.org/10.1016/j.foreco.2010.07.054]
-
Prieto, P, Penuelas, J, Llusia, J, Asensio, D, Estiarte, M., (2009), Effects of long-term experimental night-time warming and drought on photosynthesis, Fv/Fm and stomatal conductance in the dominant species of a Mediterranean shrubland, Acta Physiol Plant, 31, p729-739.
[https://doi.org/10.1007/s11738-009-0285-4]
-
Reich, PB, Sendall, KM, Stefanski, A, Rich, RL, Hobbie, SE, Montgomery, RA., (2018), Effects of climate warming on photosynthesis in boreal tree species depend on soil moisture, Nature, 562, p263-267.
[https://doi.org/10.1038/s41586-018-0582-4]
-
Sala, A, Piper, F, Hoch, G., (2010), Physiological mechanisms of drought-induced tree mortality are far from being resolved, New Phytol, 186, p274-281.
[https://doi.org/10.1111/j.1469-8137.2009.03167.x]
-
Sevanto, S, McDowell, NG, Dickman, LT, Pangle, R, Pockman, WT., (2014), How do trees die? A test of the hydraulic failure and carbon starvation hypotheses, Plant Cell Environ, 37, p153-161.
[https://doi.org/10.1111/pce.12141]
-
Tang, B, Yin, C, Wang, Y, Sun, Y, Liu, Q., (2016), Positive effects of night warming on physiology of coniferous trees in late growing season: leaf and root, Acta Oecol, 73, p21-30.
[https://doi.org/10.1016/j.actao.2016.02.002]
- Walther, GR, Post, E, Convey, P, Menzel, A, Parmesan, C, Beebee, TJ, Fromentin, J, Hoegh-Guldberg, O, Bairlein, F., (2002), Ecological responses to recent climate change, Nature, 416, p389-395.
-
Yin, HJ, Liu, Q, Lai, T., (2008), Warming effects on growth and physiology in the seedlings of the two conifers Picea asperata and Abies faxoniana under two contrasting light conditions, Ecol Res, 23, p459-469.
[https://doi.org/10.1007/s11284-007-0404-x]
-
Yun, SJ, Han, S, Han, SH, Lee, SJ, Jung, Y, Kim, S, Son, Y., (2014), Open-field experimental warming and precipitation manipulation system design to simulate climate change impact, J Korean For Soc, 103, p159-164, (In Korean with English abstract).
[https://doi.org/10.14578/jkfs.2014.103.2.159]
-
Yun, SJ, Han, S, Han, SH, Kim, S, Li, G, Park, MJ, Son, Y., (2016), Short-term effects of warming treatment and precipitation manipulation on the ecophysiological responses of Pinus densiflora seedlings, Turk J Agric For, 40, p621-630.
[https://doi.org/10.3906/tar-1511-68]
- Zhao, C, Liu, Q., (2008), Growth and photosynthetic responses of two coniferous species to experimental warming and nitrogen fertilization, Can J For Res, 39, p1-11.